Abstract
Mokala National Park (MoNP) has a history of arboricide use through South African National Parks (SANPs) having bought commercial game farmland for its establishment in 2007. Tebuthiuron arboricide is known to have been applied for controlling bush densification during the period 1996 to 2004. Persistent negative impacts on MoNP vegetation, which are ascribed to the historical arboricide use, have prompted this investigation from 2016 to 2017. Bioassay experiments employing as test plants the tree species Vachellia erioloba and Vachellia tortilis, the shrub species Senegalia mellifera and the grass Tragus berteronianus were conducted in a glasshouse. Growth responses of these species were assessed upon their exposure to a tebuthiuron concentration range that simulated expected levels in MoNP soil soon and long after application. Chemical analysis as well as bioassay with the test species Lycopersicon esculentum (tomato) were performed on soil samples collected from three depths (0−30, 30−60 and 60−90 cm) of the soil profile at two sites in MoNP where tebuthiuron was applied in the past. The three woody test species showed differential, negative growth response to tebuthiuron, and even growth of the grass species (T. berteronianus) was affected at the higher concentrations. Evidence provided by the tomato bioassay and analysis performed on soil samples collected in situ points to the putative presence of tebuthiuron, more than a decade after the last use of arboricides for controlling bush densification.
Conservation implications: If the reported evidence of the presence of phytotoxic residue of tebuthiuron in soil of MoNP would be substantiated through further research, such findings could at least partly explain the failure of natural recruitment of vegetation in those areas where the woody component was degraded because of arboricide application more than a decade ago.
Keywords: arboricide; bush densification; ecosystems; environment; herbicide residues; problem plants; soil contamination.
Introduction
It is generally accepted that dense stands of shrubs and trees can suppress herbaceous vegetation, especially grasses (Harmse, Kellner & Dreber 2016; O’Connor & Crow 1999; Smit 2005). The main concern about ‘densification’ of trees, or an undesirably dense woody component of vegetation, is in relation to reduction in grazing capacity and loss of land productivity in commercial farming settings. On game and livestock farms, in addition to its impact on grazing capacity, dense stands of the shrub Senegalia mellifera (Vahl) Seigler & Ebinger subsp. detinens (common name: black thorn; ‘swarthaak’) can be the bane of wildlife, livestock and humans alike, because of its ability to physically restrict access and mobility of humans and animals alike (Ward 2005). Thorns impart formidable defence against most herbivores, and this characteristic is also responsible for the notoriety of S. mellifera as an obstruction or hindrance on farmland and for hunters. Of course, what is considered ‘undesirably dense’ or ‘problem plant’ by some (e.g. farmers and hunters) is a valuable food and ideal habitat for the black rhinoceros (Buk & Knight 2010) and other browsers. An increase in abundance of certain shrubs and trees is commonly experienced when land is dedicated to commercial production of the game and/or livestock (Smit 2005) − the phenomenon is referred to as bush ‘densification’ or ‘thickening’ (La Grange 2010). The terminology ‘bush encroachment’ is inter alia used by O’Connor and Crow (1999) to signify ‘invasion’ of grasslands and ‘thickening’ of savanna by indigenous woody species. Its prolific growth habit is responsible for the classification of S. mellifera as ‘problem plant’ or ‘bush encroacher’, although it must be emphasised that such terminology with negative connotation is used in relation to human perceptions based on perceived threats to man’s aspirations.
Most savanna ecosystems in southern Africa, and especially semi-arid savanna, such as that which predominates in Mokala National Park (MoNP), are water limited and, consequently, increased abundance of woody species typically results in the suppression of herbaceous layer (which include forbs and grass species) (Harmse et al. 2016). S. mellifera has a shallow root system with extensive horizontal spread, in addition to a deep taproot, which makes it more competitive for water and nutrients than most other species, and hence explains its tendency to dominate plant communities of semi-arid savanna (Adams 1967; Donaldson 1967; Smit 1999). Reduction of grazing capacity is the inescapable consequence of unnatural bush densification that is brought on mainly by mismanagement of land by humans. To rectify both historical and more recent mistakes responsible for disruption of ecological balance in natural vegetation, the human solution is to institute control measures for the thinning or, even eradication, of the woody element. Chemical and mechanical methods of control are basically the only options available to deal with bush encroachment and/or densification. Mechanical control involves cutting and/or uprooting of plants, which is time-consuming and demands high levels of energy inputs, and effects are of limited duration in the case of cutting. The chemical option, which involves the use of arboricides, is by far the most economical in terms of both time and money. The glaring drawback of arboricides used for the control of woody species is that they lack selectivity in their effects on trees, shrubs and forbs, and they are also not innocuous towards grasses (Du Toit & Sekwadi 2012; WSSA 2014). Basically, all dicotyledonous species and even grasses under certain conditions, particularly at the juvenile stage, will be susceptible to arboricides that are supposed to target only certain woody species. Dosage of the arboricide, level of exposure and susceptibility of species are important determinants of eventual effects. Selectivity of arboricides can be improved or directed through the way in which they are applied. Application of arboricides by hand or by knap-sack sprayer is far more selective than aerial application by aircraft, but the latter method is far more economical than the former methods, and therefore, aerial application is the most common way in which arboricides are applied for the control of bush densification in South Africa. An often overlooked or ignored negative characteristic of arboricides is their relatively long persistence in soil. Persistence or half-life of arboricides in soil is typically measured in months and even years (WSSA 2014), with dosage and frequency of use often the main determinants of their persistence in the environment.
The Department of Environmental Affairs (DEA) publishes regulations pertaining to alien and invasive species (AIS) in terms of the National Environmental Management Biodiversity Act (NEMBA), which was promulgated on 01 October 2014. Regulation 16A of NEMBA deals with ‘indicators of bush encroachment’, that is indigenous species that are not a problem per se but are considered symptomatic (indicative) of poor land management. The NEMBA Regulation 16A lists 62 indigenous tree and 16 shrub species as ‘indicators of bush encroachment’ (Bromilow 2018). S. mellifera appears in the NEMBA Regulation 16A list, and its control with specific herbicides (arboricides) is authorised in the following provinces: Gauteng, Limpopo, Mpumalanga, Northern Cape, and North-West (Bromilow 2018).
Arboricides that are currently registered in South Africa for the control of S. mellifera are marketed under various product names, but all contain at least one of the following active ingredients: bromacil and tebuthiuron (CropLife South Africa 2016). It is on record that at least one tebuthiuron-containing product (Molopo 200 GG) has apparently been used during the period 1996 to 2004 for the control of woody species (particularly S. mellifera) on a commercial game farm that was acquired by South African National Parks (SANParks) to proclaim MoNP in 2007 (Bezuidenhout, Kraaij & Baard 2015b). Tebuthiuron is an urea-type, non-selective herbicide that controls broadleaf (including woody) plants at relatively low application rates and controls nearly all species at high rates. Tebuthiuron (e.g. MOLOPO 500 SC) is used for ‘bush control’ where woody species dominate. Prior to 2003, that game farm was dedicated to livestock production. Mokala National Park is located (29° 10’ 20.7’’ S, 24° 21’ 00.5’’ E) in the Northern Cape province, about 80 km from the city of Kimberley. At the time of it being a farming enterprise, the landowners had used chemical (arboricide) and mechanical methods for the control and, even eradication, of prolific shrubs (main target species S. mellifera) in certain areas on the farm during the period 1996 to 2004. The method of application of Molopo 200 GG product varied among areas on the farm – plant-specific application was done at a dosage of 6 kg/ha while application by aeroplane was done at a dosage of 3 kg/ha. An unknown number of treatments were done on different occasions during the period 1996 to 2004, mostly in the spring (Bezuidenhout et al. 2015b).
Bromacil and tebuthiuron arboricides have inherently long persistence in soil, and their half-life in soil is measured in months when applied once at recommended dosages, but when overdosing occurs or when used at high frequency and/or multiple applications, residues can remain biologically active in soil for several years (Cox 1998; Du Toit & Sekwadi 2012; Moore, Van Niekerk & Knight 1985; Van Eck & Van Lill 2008; WSSA 2014). In the case of tebuthiuron, it is reported that phytotoxic residue was present in soil for at least 8 years following application of this arboricide for the control of the encroacher shrub Seriphium plumosum (Du Toit & Sekwadi 2012). Residual effects of tebuthiuron are reported to be long-lasting; in areas that receive 1000 mm to 1500 mm of annual rainfall, the half-life of tebuthiuron is 12–15 months (WSSA 2014). In areas with lower rainfall, the persistence can be longer. Microbial action is primarily responsible for the degradation of tebuthiuron. A common characteristic of arboricides is their high molecular stability in the environment, and therefore, phytotoxic activity remains intact, that is persist, for months or even years, especially under dry soil conditions, near-neutral and alkaline soil pH, and low soil microbial activity (WSSA 2014). Those soil conditions are typical of semi-arid savanna ecosystems such as MoNP. Rainfall in MoNP occurs mainly in the summer months, but it is erratic and drought a common occurrence. Annual rainfall ranges from 304 mm to 622 mm (mean 415 mm) per annum. Geographical features of MoNP include rocky hills, sandy plains with underlying calcrete, plains of exposed calcrete, degraded old lands and drainage lines (Bezuidenhout et al. 2015b).
The present study aimed to determine whether tebuthiuron arboricide residues could be responsible for the persistent impacts on vegetation, which were first reported by Bezuidenhout et al. (2015b), in those areas of MoNP treated with tebuthiuron arboricide more than a decade ago. Specific objectives were to (1) follow the bioassay approach for the detection of phytotoxic residues of tebuthiuron in different depths of the Hutton soil form profile (Soil Classification Working Group 1991), (2) assess the response and relative susceptibility of two trees (protected Vachellia erioloba and Vachellia tortilis) and one shrub (S. mellifera) species to tebuthiuron and (3) determine how a grass species responds to tebuthiuron.
Research methods and design
General
Mokala National Park is situated about 70 km southwest of the city of Kimberley in the Northern Cape province MoNP GPS: 29° 15’ 5.19” S 24° 14’ 12.16” E to 28° 59’ 56.90” S 24° 23’ 8.29” E. The Puntberg (PB) soil collection site is in the western section adjacent to Puntberg (GPS: 29° 7’ 41.93” S 24° 18’ 38.00” E). The soil is deep (> 2.0 m), well-drained, aeolian sand (Hutton soil form) that was deposited on a hard calcrete layer which forms a water basin (SRK 2009) for V. erioloba (Camel thorn tree) as well as other associated plant species to grow in, the same habitat description applies for the Windpomp (WP) site (GPS: 29° 5’ 44.38” S 24° 22’ 23.65” E), which is situated on the northern side of Puntberg (Figure 1).
 |
FIGURE 1: Landscape units of Mokala National Park with locations of Puntberg and Windpomp soil sampling sites indicated |
|
Experiments with potted soil collected from two locations (Puntberg (PB) and Windpomp (WP) in MoNP (refer map in Figure 1) were conducted in a glasshouse at Hatfield Experimental Farm, University. Soil samples were collected in 2014 from the 0 cm – 30 cm, 30 cm – 60 cm and 60 cm – 90 cm depths in the soil profile at locations PB and WP and subjected to bioassay with various test species for determination of the presence of biologically active (phytotoxic) residues of tebuthiuron.
The PB and WP locations were chosen for investigation because both locations occur in those areas of MoNP where it is known that tebuthiuron and other control methods were applied as described by Bezuidenhout et al. (2015b). Bezuidenhout et al. (2015a) describe both PB and WP habitat as deep (> 1.2 m) well-drained red sandy (less than 6% clay content in A-horizon and less than 15% in B-horizon) soil. It is restricted to the undulating plains, which are overlain by aeolian sand covering the Dwyka Formation. Surface limestone occurs sporadically in this woodland and shrubland landscape units. No rocks or stones were noted on the soil surface. The dominant soil form was Hutton, while Clovelly and Mispah soil forms were also found (Soil Classification Working Group 2015). At the PB site, the landscape is closely associated with the undulating plains of V. erioloba-V. tortilis open woodland. The WP site is on the interface between the two landscape units, namely the flat plains landscape unit of S. mellifera-V. erioloba open to closed woodland, while the other landscape unit is associated with slightly undulating footslopes/midslopes of S. mellifera–V. tortilis open shrubland (Figure 1) (Bezuidenhout et al. 2015b). Selected soil properties of samples collected at PB are presented in Table 1. Soil analysis was done by Intertek Agricultural Services, Johannesburg, South Africa. Samples from WP site have similar properties as that of PB site, and therefore, only samples from the latter site were analysed for certain characteristics (Personal communication: Dr Hugo Bezuidenhout, SANParks, Kimberley).
TABLE 1: Selected properties of the Hutton soil form samples collected from three depths (cm) of the soil profile at the Puntberg location in Mokala National Park. (Analyses were performed by Intertek Agricultural Services). |
Treatments (see next section for detail) in the bioassay experiments were replicated three times and pots were arranged in a completely randomised design. All statistical analyses were performed in R v.2.8.1. Analyses of variance (ANOVA) were used to detect significant differences between and within treatment groups.
For chemical detection of tebuthiuron in all three depths of the soil profile that were sampled in 2014 at both locations, sub-samples were sent to the laboratory of RPS Mountainheath Ltd, United Kingdom, for analysis using chromatographic techniques (Smith, Shuman & Lokey 1984; Wells, Michael & Neary 1984).
Bioassay experiments
Tree and shrub test species
The tree species V. tortilis, V. erioloba and the shrub species S. mellifera were grown from seed collected in MoNP. Seedlings were transplanted into soil treated with tebuthiuron to achieve a concentration range that simulates extremely low to low residual concentrations, which conceivably, might be present in MoNP soil for several months, and even years, after application of recommended dosages of this tebuthiuron arboricide. Soil used in this experiment had to be free of any arboricide residues, therefore collection was done from the 0 cm – 30 cm soil depth of a control area of the PB site where tebuthiuron application had never been done in the past (Bezuidenhout et al. 2015b).
Because arboricides do not affect only woody species, it was decided to monitor the presence of any other plant species that would spontaneously establish on soil in this experiment. All other species that emerged and grew spontaneously with the three transplanted woody species would be exposed to the same range of tebuthiuron concentrations serving as treatments in this experiment.
Tebuthiuron was sourced from Villa Crop Protection (Pty) Ltd in the form of the product TEBUTHIURON 500 SC.
Selection of the tebuthiuron dosage range used in this experiment was based on the following measurements, assumptions and calculations:
Specific weight of Mokala soil = 1.5 g/cm3, therefore,
1 ha soil 10 cm deep (109 cm3) weighs 1.5 × 109 g, therefore,
1 m2 area soil 10 cm deep weighs 1.5 × 102 kg.
MOLOPO 500 SC label recommendation (500 g active ingredient L-1):
Stock solution: 1.5 L product in 8.5 L water (= 750 g in 10 L stock sol; = 75 mg/mL).
Apply 8 mL (4 × 2-mL dosage stock sol) at base of 2-m tall multi-stemmed tree, therefore,
8 × 75 mg = 600 mg tebuthiuron, therefore,
assume 600 mg tebuthiuron is applied per 1 m2 soil surface area, and it is uniformly distributed 10 cm deep, therefore,
600 mg tebuthiuron per 1.5 × 102 kg soil, therefore,
1 kg soil contains 4 mg tebuthiuron [= 4 mg/kg (ppm) tebuthiuron in 1 m2 area, 10 cm deep];
[= 0.4 mg/kg tebuthiuron in 1 m2 area, 100 cm deep].
Based on the above assumptions and calculations, tebuthiuron treatments applied on 06 February 2017 comprised the following dosage range: 0 mg/kg (untreated control), 0.005, 0.01, 0.05, 0.1, 0.5 mg/kg. Treatment was done by applying predetermined volumes of 10 000-times diluted stock solution, which were prepared from the commercial product, in 80 mL total volume (made up with pure water) per pot containing 800 g soil.
Seed of V. erioloba, V. tortilis and S. mellifera were collected in MoNP on 08/09 January 2017. For promotion of germination, seeds of V. erioloba and V. tortilis was scarified on sanding paper prior to soaking in warm water overnight – temperature of water was 70 °C at immersion of seeds, with no further heating of water. Seeds of S. mellifera was simply immersed overnight in water at room temperature (25°C – 28°C). Following soaking in water, seeds were placed on, and covered with, moist paper towels to allow germination at room temperature. After 7 days, germination had occurred to the extent that it was possible to select germinated seeds from which the radicle extended at least 5 mm. Seedlings were transplanted to pots containing 800 g MoNP soil sampled near the PB locality in an area that do not have a history of tebuthiuron application on 20 January 2017.
Water was added to soil in pots to attain field capacity level at the onset of the experiment. Following treatment with tebuthiuron, total mass of each experimental unit (pot with soil) comprising pot, soil, seedlings and water was measured by weighing, and total mass recorded for each pot. Subsequently, pots were weighed twice a week to replenish as accurately as was practically possible water lost through evaporation and transpiration. Each week a total volume of 100 mL of a commercial complete nutrient solution (Multifeed) was applied to each pot to prevent the risk of nutrient deficiencies confounding the results.
The experiment was terminated when herbicide effects were judged to have reached maximum level. Visual estimate of % damage of total leaves affected (expressed as percentage damage) caused by tebuthiuron was made with 2-week (14-day) intervals, which commenced 14 days after treatment (DAT). The final measurement (visual assessment of damage expressed as percentage of the control) was done 56 days after herbicide application, and the experiment was terminated at 60 DAT.
Statistical analysis employed the R v.2.8.1 programme. For the tree and shrub species, the amount of damage was compared across locations, species and soil depth at different treatment levels using the multifactor ANOVA approach.
Common carrot-seed grass (Tragus berteronianus)
A single plant species was observed to establish spontaneously at all treatments in the experiment discussed above, namely the pioneer annual grass Tragus berteronianus. Its presence was monitored at different treatments and growth compared between tebuthiuron treatments and the control treatment (zero tebuthiuron). Arboricide effect on this grass species was measured through visual assessment of growth reduction at treatments relative to growth at the control treatment. Statistical analysis of data was done with the R v.2.8.1 programme. Damage over time was compared between the different treatments with a simple ANOVA.
Tomato (Lycopersicon esculentum Mill.) test species
Seed of cultivar Heinz 1370 was sown on soil collected from three soil depths (0 m – 30 m, 30 m – 60 m and 60 cm – 90 cm) at both PB and WP locations where tebuthiuron had been applied in the past (Bezuidenhout et al. 2015b). Soil samples serving as untreated controls were extracted from the three soil depths by means of a soil auger at both sites in those areas where tebuthiuron was not applied at any stage in the past (control sites). Six seeds were sown in each pot, and following emergence, seedlings were thinned to three per pot. Seedling height and aboveground fresh biomass were measured at termination of the experiment, that is 30 days after sowing of seed. Water and nutrient solution were applied in the same manner and frequency as that described above for the ‘Tree and shrub test species’ experiment. Data were expressed as mean plant height and biomass per pot prior to statistical analysis performed with R v.2.8.1. Height and biomass were compared between locations and depths using a multifactor ANOVA.
Chemical analysis for detection of arboricide residues in soil samples
Soil analysis was conducted by RPS Mountainheath Ltd (http://www.rpsgroup.com), United Kingdom, and was focused on tebuthiuron in all soil samples. Analyses results are presented in Table 2 in the following section.
TABLE 2: Tebuthiuron presence in soil samples from different depths at Puntberg and Windpomp sites in Mokala National Park. (Minimum detection level was set at 0.1 mg/kg). |
Results
Bioassay experiments
Tree and shrub test species
Seedling growth response, which was expressed as estimated damage percentage based on visual comparison of tebuthiuron-treated seedlings of V. erioloba, V. tortilis and S. mellifera with their respective controls (untreated seedlings), appear in Figure 2. Averaged across tebuthiuron concentration, damage was already visible at 14 DAT in the form of symptoms, which manifest as chlorosis on leaves, that are typical of a photosynthesis-inhibiting arboricide (tebuthiuron in this case). The severity of damage symptoms, that is, the intensity of chlorosis increased with tebuthiuron concentration and time. No damage symptoms caused by tebuthiuron were observed on any of the seedling types at 0.005 and 0.01 mg/kg concentrations for the duration of the experiment (56 DAT).
 |
FIGURE 2: Growth reduction (% damage) calculated across a range of tebuthiuron concentrations that were established in soil collected from site Puntberg in an area which did not have a history of tebuthiuron application. Observations for which data are presented here were made 14 days after treatment (14 DAT –‘Day a’), 28 DAT (‘Day b’) and 56 DAT (‘Day c’) (Erioloba – Vachellia erioloba, Mellifera – Senegalia mellifera, Tortilis – Vachellia tortilis). |
|
Over the 14 DAT to 56 DAT period S. mellifera and V. erioloba showed a significant increase in damage beyond 28 DAT. V. tortilis showed a significant increase in damage earlier on between days 14 and 28. All three species showed a similar tendency for increased damage with time (Figure 2). In all three species, leaf senescence and shedding of leaves followed the manifestation of chlorosis on leaves, but the former symptoms (leaf senescence) manifested only at the relatively high tebuthiuron concentrations of 0.1 mg/kg and 0.5 mg/kg. Of the three species, across tebuthiuron concentration and time, V. tortilis tended to be the most susceptible to this arboricide, with V. erioloba and S. mellifera showing lower and similar tolerance to tebuthiuron.
Common carrot-seed grass (Tragus berteronianus)
The effect of tebuthiuron arboricide on the growth of this grass species could be assessed because of the presence of seeds of T. berteronianus occurring naturally in the soil collected for bioassay from MoNP. Tebuthiuron effects, which were expressed as damage percentage, were measured by visual comparison of the grass’ growth and development at tebuthiuron treatments and the untreated control (Figure 3). Damage symptoms that were visible as leaf chlorosis and failure of seedling emergence were observed at tebuthiuron concentrations of 0.05 mg/kg, 0.1 mg/kg and 0.5 mg/kg, and symptoms increased significantly with an increase in concentration across this higher end of the concentration range. Relatively low percentage damage was already observed at 14 DAT and damage increased significantly over time. Failure of seedling emergence was the common observation at the maximum concentration of the arboricide.
 |
FIGURE 3: Growth reduction (% damage) caused to the grass species Tragus berteronianus by different tebuthiuron concentrations that were established in untreated (control) soil collected at site Puntberg. Observations for which data are presented here were made 14 days after treatment (14 DAT – ‘Day a’), 28 DAT (‘Day b’) and 56 DAT (‘Day c’). |
|
Tomato (Lycopersicon esculentum L.) test species
In this experiment, the soil in bioassay was not treated with tebuthiuron or any other arboricide and was sourced from three depths (0 cm − 30 cm; 30 cm − 60 cm and 60 cm − 90 cm) in the soil profile of the two sites PB and WP that have a history of arboricide use, that is, tebuthiuron according to available information (Bezuidenhout et al. 2015b). A third site near the aforementioned PB site does not have a history of arboricide application and consequently served as ‘control’, that is, soil not contaminated with arboricide residues. Growth of tomato seedlings at different treatments was measured by harvesting and weighing of seedlings when the experiment was terminated. Seedling weight in gram per pot (treatment combination) appears in Figure 4.
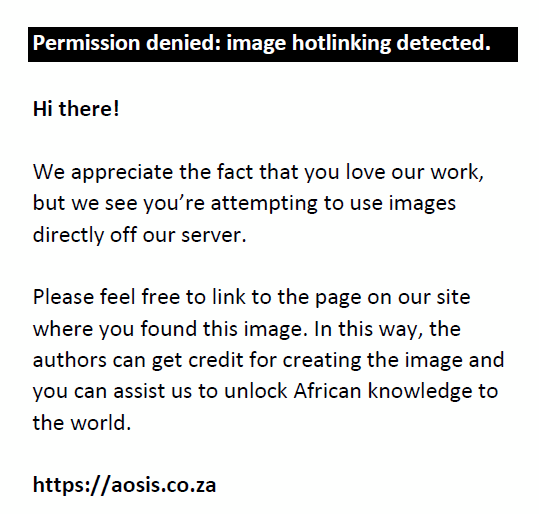 |
FIGURE 4: Growth response (seedling weight) of tomato cultivar Heinz 1378 on soil collected from three depths (‘a’ = 0 cm – 30 cm; ‘b’ = 30 cm – 60 cm); ‘c’ = 60 cm – 90 cm) of the soil profile at three locations (Puntberg-West; Windpomp; untreated control). |
|
Significant reduction in tomato weight measured for soil collected from both the 0 cm − 30 cm and 60 cm − 90 cm depths in the soil profile at both the PB and WP sites (Figure 4) is indicative of the presence of a growth-inhibiting factor, such as putative phytotoxic residue(s) of an arboricide(s). Tomato growth on soil from the 30 cm − 60 cm depth of both those sites was not significantly different from growth attained on control soil and therefore is indicative of lack of a growth-inhibiting factor(s) or to the presence of factors in too low amount to cause growth reduction in the test species. None of the soil characteristics presented in Table 1 can explain the significant tomato growth reductions relative to the control treatments, which occurred on soil from specific depths, at both PB and WP sites with a history of arboricide application.
Chemical analysis for the presence of arboricide residues in soil samples
The presence of tebuthiuron arboricide was revealed by chemical analysis in all three soil depths (0−30, 30−60 and 60−90 cm) at both sites (Table 2). In all samples, the amount of tebuthiuron was found to be lower than the minimum detection level (MDL), which means it was detected but could not be accurately quantified. The MDL applied by the laboratory for these analyses, that is, 0.1 mg/kg, which is the same concentration as one of the tebuthiuron treatments that caused phytotoxic plant responses (damage symptoms) on test plants in the bioassays with tree, shrub and the grass species.
Discussion
Commercial products of arboricides such as Molopo 200 GG, in which the active ingredient is tebuthiuron, represent one method (chemical) used for the control of woody species on the commercial livestock and game farm that was acquired by SANParks to establish MoNP in 2007. Bezuidenhout et al. (2015b) document the history of arboricide applications and other vegetation management practices and contend that impacts of these treatments on vegetation in MoNP have persisted for more than a decade. According to Bezuidenhout et al. (2015b), the arboricide tebuthiuron was applied in granular formulation (Molopo 200 GG product) on said commercial farm in the period 1996 to 2004 for the control of the woody plant species S. mellifera and V. tortilis. Because tebuthiuron is nonselective in terms of effect on all dicotyledonous species, more herbaceous and woody species would succumb to this arboricide than just the main target species, S. mellifera.
In the present study, all three the woody species assessed, viz. V. tortilis, V. erioloba and S. mellifera, were susceptible (showed damage symptoms) to effects of tebuthiuron at 0.1 mg/kg and 0.5 mg/kg concentrations when exposed at the seedling stage. These concentrations could conceivably be relevant to tebuthiuron levels present in soil following application of recommended dosages of a product such as Molopo 200 GG for ‘bush control’ or ‘controlling encroacher species’, that is, for killing mature woody plants. It should be considered that under natural conditions, over a prolonged period of months and even years, relatively low, non-phytotoxic arboricide concentrations in soil can be taken up by plants and progressively accumulate to phytotoxic amounts in the plant system and eventually cause damage to plants long after initial arboricide application. As an example of herbaceous species’ response to tebuthiuron, the tomato test species used in this study proved to be an effective indicator of the presence of phytotoxic residues of tebuthiuron in MoNP soil (Figure 4). The bioassay technique that we used is generally accepted to be effective for determining the presence of plant-damaging (phytotoxic) residues of herbicides, including arboricides, in soil.
The presence of bioactive residues of tebuthiuron in the 0 cm − 90 cm soil profile of the two sites monitored in MoNP was confirmed by chemical analysis (Table 2).
Arboricides used for the control of woody species are generally considered to be selective towards grass species, that is, grass species are expected to be impervious to arboricides but then only under specific circumstances (Du Toit & Sekwadi 2012). At dosage rates significantly higher than field-use recommended rates, arboricides can damage all types of vegetation, especially herbaceous and woody species, and even grasses can be damaged or killed when exposed to excessive amounts of arboricides (Cobb & Reade 2010). We observed that the grass species T. berteronianus could be severely affected by tebuthiuron depending on its concentration in soil of MoNP (Figure 3), even to the extent that the emergence of seedlings is prevented. This points to the possibility that recruitment of grasses in general can be curtailed and even prevented when seeds are exposed to relatively high concentrations of an arboricide such as tebuthiuron. Du Toit and Sekwadi (2012) report that tebuthiuron remains biologically active for at least 8 years after application in semi-arid grassland (Cymbopogon-Themeda veld type). They used the bioassay technique with oats (Avena sativa) and cabbage (Brassica oleracea) as indicator species for the presence of tebuthiuron in soil to explain the development of bare patches in the grass layer where this arboricide was applied for the control of the encroacher shrub Seriphium plumosum. Bauer (1978) found that tebuthiuron in either liquid or granular formulation prevented ryegrass (Lolium perenne) establishment when applied at the 3.4 kg/ha dosage rate 261 days before planting of this grass pasture. Picloram arboricide applied after the emergence of Bermuda grass (Cynodon dactylon) temporarily reduced the yield of this grass, and yield recovery only occurred the following season (Bauer, Bovey & Holt 1977). Generally, the grass component of areas that previously was dominated by dense stands of S. mellifera is reported to show good long-term recovery after the dominant woody species are killed by arboricides (Moore et al. 1985), but there is a scant in-depth research on how different grass species respond to specific arboricides in semi-arid environments. Just as woody species differ in their susceptibility to arboricides, grasses are likely to respond differently as well.
Bezuidenhout et al. (2015b) advise consideration of active rehabilitation measures if the natural recovery in the arboricide-treated areas of MoNP proves to be inadequate to ensure the conservation of ecological pattern and process. They contend that phytotoxic residues of tebuthiuron arboricide present in soil could be impacting vegetation recruitment even 10 years after this arboricide was applied. Key edaphic factors that prolong the period for which arboricides can remain biologically active (phytotoxic) in soil are the following: low rainfall, near-neutral soil pH, low microbial activity, low clay content in soil profile and low organic carbon content of soil (WSSA 2014). Dosage and frequency of use remain key drivers of the persistence of arboricides in soil. The aforementioned long periods of impact following their use are likely to occur only if excessive dosages and/or high frequency of use were the historical practice. In the case of MoNP, it appears that the records of historical arboricide application and current contamination levels are incomplete, and only through further investigation can the real situation be revealed. We contend that only once such information is available can vegetation rehabilitation efforts have a high probability of being successful.
Conclusions
Although conclusive proof is lacking at this stage, both the bioassay approach and chemical analysis in the present investigation revealed the presence of phytotoxic residue of the arboricide tebuthiuron in soil samples collected from sites in MoNP with a history of application of this herbicide for controlling bush densification. Effects of tebuthiuron observed on seedlings of V. erioloba, V. tortilis and S. mellifera suggest that recovery of the woody species following tebuthiuron application, especially at relatively high dosage rates and high frequency, for the control of mature plants could impede or even prevent the establishment of seedlings or saplings of those species for a significant period post-use. The establishment of grass species could also be impacted by tebuthiuron, as shown by the response of T. berteronianus in our bioassay experiment. Soil and environmental conditions in MoNP favour the long persistence (half-life) of arboricides such as tebuthiuron and probably explain the apparent excessively long persistence of tebuthiuron in this setting. Many key drivers of the long persistence of arboricides in soil are characteristic of semi-arid savanna areas of southern Africa where arboricide use for controlling bush densification is often common practice, and unfortunately, excessive in terms of various arboricide combinations, high dosage rates and high frequency of application. For any rehabilitation effort of affected areas in MoNP to have a reasonable chance of success, further investigation on the nature and extent of soil contamination with arboricide residues is imperative.
Acknowledgements
Competing interests
The authors declare that they have no financial or personal relationships that may have inappropriately influenced them in writing this article.
Authors’ contributions
C.R. and H.B. were responsible for conceptualisation. H.B. was the project leader and contributed to the sampling of soil and seeds in Mokala National Park. C.R. was the main writer and was responsible for the experiments. C.R. and H.B. organised the resources. J.B. conducted statistical analyses and produced graphs based on the data that was collected by C.R. and contributed towards describing the methods. All authors contributed to the final drafting of the manuscript.
Ethical considerations
This article followed all ethical standards for research without direct contact with human or animal subjects.
Funding information
SANParks and Mokala National Park Management provided technical assistance for the field work, and provided accommodation for the researchers. The University of Pretoria provided support in the form of glasshouse facilities, and Villa Crop Protection (Pty) Ltd provided partial financial support.
Data availability
Data sharing is not applicable to this article as no new data were created or analysed in this study.
Disclaimer
The views and opinions expressed in this article are those of the authors and do not necessarily reflect the official policy or position of any affiliated agency of the authors.
References
Adams, M.E., 1967, ‘A study of the ecology of Acacia mellifera, A. seyal and Balanites aegyptica in relation to land-clearing’, Journal of Applied Ecology 4(1), 221–237. https://doi.org/10.2307/2401420
Bauer, J.R., 1978, ‘Effects of picloram and tebuthiuron on establishment on ryegrass winter pasture’, Journal of Range Management 31(6), 450–455. https://doi.org/10.2307/3897205
Bauer, J.R., Bovey, R.W. & Holt, F.C., 1977, ‘Effect of herbicides on production and protein level in pasture grasses’, Agronomy Journal 69(5), 846–851. https://doi.org/10.2134/agronj1977.00021962006900050030x
Bezuidenhout, H., Bradshaw, P.L., Bradshaw, M. & Zietsman, P.C., 2015a, ‘Landscape units of Mokala National Park, Northern Cape Province, South Africa’, Navorsinge van die Nasionale Museum Bloemfontein 31(1), 1–27.
Bezuidenhout, H., Kraaij, T. & Baard, J., 2015b, ‘Persistent effects of chemicals used to control shrub densification in semi-arid savanna’, Earth Science Research 4(1), 31–39. https://doi.org/10.5539/esr.v4n1p31
Bromilow, C., 2018, Problem plants and invasive weeds of southern Africa, Briza Publications, Pretoria.
Buk, K.G. & Knight, M.H., 2010, ‘Seasonal diet preferences of black rhinoceros in three arid South African National Parks’, African Journal of Ecology 48(4), 1064–1075. https://doi.org/10.1111/j.1365-2028.2010.01213.x
Cobb, A.H. & Reade, J.P.H., 2010, Herbicides and plant physiology, 2nd edn., Wiley-Blackwell, UK.
Cox, C., 1998, ‘Picloram, Herbicide Fact Sheet’, Journal of Pesticide Reform 18(1), 13–20.
CropLife South Africa, 2016, Problem plant control compendium, AVCASA, Halfway House.
Donaldson, C.H., 1967, Bush encroachment with special reference to the Black thorn problem of the Molopo area, Department of Agricultural Technical Services, Armoedsvlakte Research Station, Vryburg.
Du Toit, J.C.O. & Sekwadi, K.P., 2012, ‘Tebuthiuron residues remain active in soil for at least eight years in a semi-arid grassland, South Africa’, African Journal of Range & Forage Science 29(2), 85–90. https://doi.org/10.2989/10220119.2012.705325
Harmse, C.J., Kellner, K. & Dreber, N., 2016, ‘Restoring productive rangelands: A comparative assessment of selective and non-selective chemical bush control in a semi-arid Kalahari savanna’, Journal of Arid Environments 35, 39–49. https://doi.org/10.1016/j.jaridenv.2016.08.009
La Grange, M., 2010, ‘Vegetation classification of the proposed Heritage Park, North-West Province, South Africa’, MSc thesis (Environmental Sciences), North-West University, Potchefstroom.
Moore, A., Van Niekerk, J.P. & Knight, I.W., 1985, ‘The effect of tebuthiuron on the vegetation of the thorn bushveld of the Northern Cape – A preliminary report’, Journal of the Grassland Society of South Africa 2(4), 7–10. https://doi.org/10.1080/02566702.1985.9648012
O’Connor, T.G. & Crow, V.R.T., 1999, ‘Rate and pattern of bush encroachment in Eastern Cape savanna and grassland’, African Journal of Range and Forage Science 16(1), 26–31. https://doi.org/10.2989/10220119909485715
Smit, G.N., 1999, Guide to the Acacias of South Africa, Briza Publications, Pretoria.
Smit, G.N., 2005, ‘Tree thinning as an option to increase herbaceous yield of an encroached semi-arid savanna in South Africa’, BMC Ecology 5(4), 15. https://doi.org/10.1186/1472-6785-5-4
Smith, A.E., Shuman, L.M. & Lokey, N., 1984, ‘High-pressure liquid chromatographic analysis of tebuthiuron in soil’, Journal of Agricultural Food and Chemistry 34, 416–418. https://doi.org/10.1021/jf00122a060
Soil Classification Working Group, 1991, ‘Soil classification: A taxonomic system for South Africa’, Memoirs on the Agricultural Natural Resources of South Africa 15, 1–262.
SRK, 2009, ‘Consulting engineers and scientists: Mokala yield testing. Internal report, Park Manager: Mokala National Park, South African National Park.
Van Eck, J.A.J. & Van Lill, C., 2008, ‘Chemical bush control on Rhigozum trichotomum and Acacia nebrownii in southern Namibia using different arboricides and control methods’, Agricola 15, 51–55.
Ward, D., 2005, ‘Do we understand the causes of bush encroachment in African savannas?’, African Journal of Range & Forage Science 22(2), 101–105. https://doi.org/10.2989/10220110509485867
Weed Science Society of America (WSSA), 2014, Herbicide handbook, 10th edn., WSSA, Lawrence, KS.
Wells, M.J.M., Michael, J.L. & Neary, D.G., 1984, ‘Determination of picloram in soil and water by reversed-phase liquid chromatography’, Archives of Environmental Contamination and Toxicology 13, 231–235. https://doi.org/10.1007/BF01055881
|